Understanding Cracks in Residential Concrete; Control and Prevention
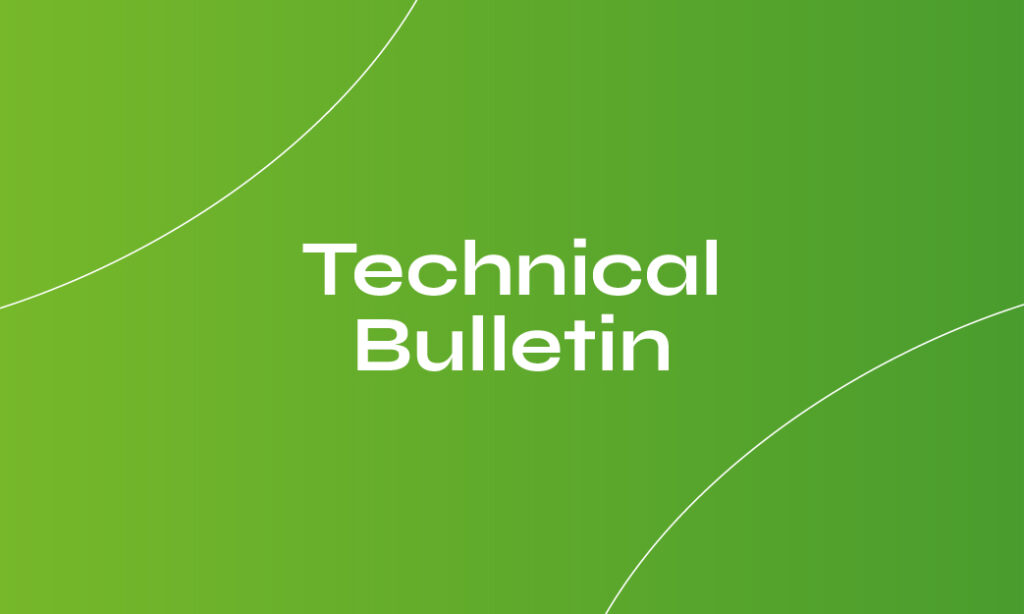
Cracks in concrete come in many sizes and shapes: from superficial but aesthetically displeasing surface cracks, to wide, full-depth cracks. This article is written for homeowners, homebuilders or building inspectors to provide a basic understanding of the most common types of cracks, their control and prevention.
Concrete Cracks: Common Types and Causes
Concrete, in its simplest form, is a mixture of hydraulic cement, aggregates and water1. The majority of concrete produced today also contains chemical admixtures such as water-reducers, retarders and accelerators. Like many other construction materials, concrete expands and contracts with changes in temperature and moisture. It may also deflect depending on applied loads and support conditions.
Have you ever seen a slab, driveway, porch or sidewalk and thought that it looked beautiful except for that ugly crack? When anything happens that causes concrete to expand or contract, or when a heavy load is applied, or when a change in support occurs, concrete will likely crack.
One of the most common forms of cracks are called plastic shrinkage cracks. Plastic shrinkage cracks appear in the surface of fresh concrete soon after it is placed and before it hardens2. They are caused by the rapid loss of water from the surface of the concrete before it hardens. These cracks are usually parallel, shallow and do not reach the edge of the slab. The addition of water to the concrete, high temperatures, low humidity, and wind speed are primary causes of plastic shrinkage cracks. Because they are shallow, they are often unsightly, but rarely impair the strength or durability of the concrete.
Drying shrinkage cracks are another common form of cracking, and are probably the most misunderstood and contentious cracks in residential concrete. When concrete is placed, it is at its largest volume. As it begins to harden, the water from inside the concrete comes to the surface and evaporates; this water is called bleed water. The loss of bleed water causes a reduction in volume and the concrete begins to shrink. Once the concrete starts to harden (through cement hydration) water is consumed in the reaction process while it continues to externally dry. This total loss of water leads to a contraction of the concrete, termed drying shrinkage, and is the primary cause of the larger, unsightly, often full-depth cracks known as drying shrinkage cracks. When the concrete is restrained from shrinking freely, due for example to thickened edges along the perimeter, internal grade beams for load-bearing walls, M-E-P rough-ins, etc., the probability and size of drying shrinkage cracks increases. Drying shrinkage is why concrete finishers place joints in the concrete; joints are simply “pretty cracks.” On average, concrete shrinks 1/8 inch in twenty feet, but in general, drying shrinkage is proportional to the amount of water in the concrete3.
Cracks may also form due to settlement of the concrete around steel (which increases in likelihood of occurrence with the addition of water), formwork movement, changes in subgrade support (such as at an improperly compacted trench) or large changes in temperature while the concrete is still in early stages of hardening2.
As referenced above, the common practice of adding water to the concrete to ease in its placing and finishing is frequently a primary cause of many of these cracks, and at a minimum contributes to making them worse. Therefore, the addition of a mid-range or high-range water reducing admixture to minimize the amount of water in the concrete would help to solve many of these problems.
Welded Wire Reinforcement (WWR)
Most residential slabs do not have primary, or structural, steel reinforcement. If steel is used, it is used as secondary reinforcement which is defined by the American Concrete Institute (ACI)4 as “nonstructural reinforcement such as (WWR), fibers or bars to minimize crack widths that are caused by thermal expansion and contraction, or shrinkage”.
The Wire Reinforcement Institute (WRI) says that drying shrinkage is the greatest concern in the design and construction of slabs-on-ground. They define three primary purposes for reinforcing slabs-on-ground: (drying) shrinkage control, temperature control and moment capacity5. For WWR to perform, it must be accurately positioned within the top third of the concrete with adequate support. One of the primary causes for under-performing residential slabs is the inadequate positioning or complete absence of supports6. Studies suggest that for light-gauge WWR commonly used in residential slabs, spacing of supports no greater than 12 inches in each direction should be provided7. Additionally, WWR does not provide for the reduction of plastic shrinkage cracking. However, there is an alternative that provides all three of the primary purposes for reinforcing a slab and control of plastic shrinkage cracks…
Synthetic Fibers
Synthetic fibers (fiber) are defined by ACI as “chopped fibers made of polyolefin, such as polypropylene and polyethylene materials, used as reinforcement in concrete”8. Over the past four decades, the use of fiber in concrete has been increasing at an exponential rate. They provide a more complete solution to cracking due to their dispersion throughout the concrete. Whereas there may be ~150 feet of steel wire located two-dimensionally at only one depth in a given volume of concrete, alternatively there are hundreds of thousands of fibers in that same volume located three-dimensionally throughout the depth of the slab. This means that for a properly fiber reinforced concrete (FRC) slab, all types of cracks can be minimized or eliminated before they can form.
ASTM C1116/C1116M defines Type III Synthetic Fiber-Reinforced Concrete as concrete that contains synthetic fibers for which documentary evidence can be produced confirming their resistance to deterioration9. There are two distinct types of synthetic fibers that can be used for FRC, micro-synthetic fibers and macro-synthetic fibers.
Micro-Synthetic Fibers
ACI10 defines microfibers as fibers with diameters less than 0.012 in (0.3mm). There are two types of microfibers, monofilament and fibrillated. These fibers are typically 0.5 to 1.5 inches in length and added to the concrete at 0.5 to 3.0 pounds per cubic yard (pcy). Monofilament microfibers are like individual strands of silk, whereas fibrillated microfibers are like a net. Fibrillated microfibers are generally thicker and wider than monofilament microfibers, and are cut in a pattern longitudinally creating a net when pulled apart. Microfibers provide superior resistance to the formation of plastic shrinkage cracks, especially when compared to WWR. They also help to improve freeze-thaw durability, and resistance to cracking and spalling. However, once a crack forms, they are unable to provide post-crack load carrying capacity and are therefore not an equivalent replacement for WWR. Fibrillated microfibers, however, when dosed at a minimum of 1.5 pcy may be used as an alternative to WWR to provide temperature and shrinkage crack control.
Macro-Synthetic Fibers
ACI8, 10 defines macrofibers as fibers with diameters greater than 0.012 in (0.3mm) and the capability to enhance the engineering properties of concrete; that is, provide performance that meets or exceeds that of WWR. These fibers are typically 1.25 to 2 inches in length and, for residential slabs, they are typically added to concrete at 3 to 5 pounds per cubic yard. Macrofibers have many shapes including tape, straw or twisted strands. They provide all of the benefits of microfibers plus increased durability and toughness, and equal or better control of crack propagation and crack width opening as compared to WWR. Simply put, macrofibers perform equivalently to WWR plus help to minimize plastic shrinkage cracks if the proper dosage is used since they are dispersed throughout the concrete.
Determining Equivalent Performance
Macrofibers can be engineered to provide performance equal to that of WWR. To determine the proper dosage rate, comparison of actual performance data can be evaluated. Different tests can be used to obtain performance data, but only ASTM C1609/C1609M11 can be used to test fibers and WWR head-to-head. The two primary test results to review are the equivalent flexural residual strength, fe3 or f(e,150)150, and the equivalent flexural residual strength ratio, Re3 or R(T,150)150. Because fe3 is influenced by the compressive strength and peak flexural strength, comparison of fe3 values between tests can be difficult. Therefore, a comparison of Re3 values is more appropriate as it relates fe3 as a percentage of the peak stress in the concrete. Accordingly, to assure that the synthetic fiber’s performance meets or exceeds that of WWR, the fiber’s Re3 value should be greater than that of WWR when tested using ASTM C1609/C1609M. The test results below, performed by an independent laboratory, provide ASTM C1609/C1609M comparison data for a microfiber, macrofiber and WWR.
Based upon this data, STRUX 90/40, a synthetic macrofiber added to concrete at a rate of 3.0 pounds per cubic yard exceeds the performance of WWR. The microfiber, although exceeding the ability of the WWR to mitigate plastic shrinkage cracks, cannot meet or exceed the performance of the WWR once a crack occurs.
References
- 1. ACI Concrete Terminology 2018 7. “The Contractor’s Welded Wire Reinforcement (WWR) Dilemma”; Malisch, Ward and Suprenant, Bruce
- 2. NRMCA CIP 5 “Plastic Shrinkage Cracking” 8. ACI 544.4R-18 “Guide to Design with Fiber-Reinforced Concrete”
- 3. ACI 224R-01 “Control of Cracking in Concrete Structures” 9. ASTM C1116/C1116M “Standard Specification for Fiber Reinforced Concrete”
- 4. ACI Education Bulletin E2-00 “Reinforcement for Concrete – Materials and Applications” 10. ACI 544.3R-08 “Guide for Specifying, Proportioning, and Production of Fiber-Reinforced Concrete”
- 5. WRI Tech Fact TF-705-R-03 “Formulas for Success: Innovative Ways to Reinforce Slabs-on-Ground” 11. ASTM C1609/C1609M “Standard Test Method for Flexural Performance of Fiber-Reinforced
Concrete (Using Beam With Third-Point Loading) - 6.WRI Tech Fact TF 702-R-08 “Low Maintenance Slabs: Supports are Needed for Long-Term Performance of Welded Wire Reinforcement in Slabs-on-Grade”